Role of Ultrasonography in Cancer Theranostic Applications
Article Information
Karunanithi Rajamanickam*, Joy Sebastian Prakash J
Faculty of Allied Health Sciences, Chettinad Academy of Research and Education, Tamilnadu, India
*Corresponding author: Karunanithi Rajamanickam, Faculty of Allied Health Sciences, Chettinad Academy of Research and Education, Kelambakkam, Kanchipuram District, Chennai- 603 103, Tamilnadu, India
Received: 24 December2019; Accepted: 07 January 2020; Published: 15 January 2020
Citation:
Karunanithi Rajamanickam, Joy Sebastian Prakash J. Role of Ultrasonography in Cancer Theranostic Applications. Archives of Internal Medicine Research 3 (2020): 032-043.
View / Download Pdf Share at FacebookAbstract
Ultrasound imaging technique involves the use of sound waves and their echoes to produce a picture of internal organs or masses. An ultrasound transducer emits sound waves and picks up the echoes as they bounce off from the body tissue interfaces. These echoes are converted into images to display on the monitor. This imaging modality is completely non-invasive, helps in real time monitoring, it is a cost-effective, portable and versatile instrument with a wide range of clinical utility including characterizing lesions in the liver, urogenital tract, various soft tissues etc. With the introduction of micro-bubbles intravascular structures are examined based on their vascularity. In the context of cancer imaging, ultrasound is used for numerous routine screening examinations; it assists x-ray mammography, it has the ability to characterize liver lesions, with respect to urogenital tract, it helps to detect non-symptomatic cancer in prostate, uterus, ovaries, bladder and kidney. Apart from these, it helps to characterize lymph nodes by its architectural changes and shape, also helps to diagnose and staging of thyroid and neck cancers. This review gives an overview of the history and the current scenario of targeted ultrasound imaging of cancers of different tissues.
Keywords
Contrast-Enhanced Ultrasonography, Breast Cancer, Prostate Cancer, Liver Cancer
Contrast-Enhanced Ultrasonography articles Contrast-Enhanced Ultrasonography Research articles Contrast-Enhanced Ultrasonography review articles Contrast-Enhanced Ultrasonography PubMed articles Contrast-Enhanced Ultrasonography PubMed Central articles Contrast-Enhanced Ultrasonography 2023 articles Contrast-Enhanced Ultrasonography 2024 articles Contrast-Enhanced Ultrasonography Scopus articles Contrast-Enhanced Ultrasonography impact factor journals Contrast-Enhanced Ultrasonography Scopus journals Contrast-Enhanced Ultrasonography PubMed journals Contrast-Enhanced Ultrasonography medical journals Contrast-Enhanced Ultrasonography free journals Contrast-Enhanced Ultrasonography best journals Contrast-Enhanced Ultrasonography top journals Contrast-Enhanced Ultrasonography free medical journals Contrast-Enhanced Ultrasonography famous journals Contrast-Enhanced Ultrasonography Google Scholar indexed journals Breast Cancer articles Breast Cancer Research articles Breast Cancer review articles Breast Cancer PubMed articles Breast Cancer PubMed Central articles Breast Cancer 2023 articles Breast Cancer 2024 articles Breast Cancer Scopus articles Breast Cancer impact factor journals Breast Cancer Scopus journals Breast Cancer PubMed journals Breast Cancer medical journals Breast Cancer free journals Breast Cancer best journals Breast Cancer top journals Breast Cancer free medical journals Breast Cancer famous journals Breast Cancer Google Scholar indexed journals Prostate Cancer articles Prostate Cancer Research articles Prostate Cancer review articles Prostate Cancer PubMed articles Prostate Cancer PubMed Central articles Prostate Cancer 2023 articles Prostate Cancer 2024 articles Prostate Cancer Scopus articles Prostate Cancer impact factor journals Prostate Cancer Scopus journals Prostate Cancer PubMed journals Prostate Cancer medical journals Prostate Cancer free journals Prostate Cancer best journals Prostate Cancer top journals Prostate Cancer free medical journals Prostate Cancer famous journals Prostate Cancer Google Scholar indexed journals Liver Cancer articles Liver Cancer Research articles Liver Cancer review articles Liver Cancer PubMed articles Liver Cancer PubMed Central articles Liver Cancer 2023 articles Liver Cancer 2024 articles Liver Cancer Scopus articles Liver Cancer impact factor journals Liver Cancer Scopus journals Liver Cancer PubMed journals Liver Cancer medical journals Liver Cancer free journals Liver Cancer best journals Liver Cancer top journals Liver Cancer free medical journals Liver Cancer famous journals Liver Cancer Google Scholar indexed journals thyroid articles thyroid Research articles thyroid review articles thyroid PubMed articles thyroid PubMed Central articles thyroid 2023 articles thyroid 2024 articles thyroid Scopus articles thyroid impact factor journals thyroid Scopus journals thyroid PubMed journals thyroid medical journals thyroid free journals thyroid best journals thyroid top journals thyroid free medical journals thyroid famous journals thyroid Google Scholar indexed journals neck cancers articles neck cancers Research articles neck cancers review articles neck cancers PubMed articles neck cancers PubMed Central articles neck cancers 2023 articles neck cancers 2024 articles neck cancers Scopus articles neck cancers impact factor journals neck cancers Scopus journals neck cancers PubMed journals neck cancers medical journals neck cancers free journals neck cancers best journals neck cancers top journals neck cancers free medical journals neck cancers famous journals neck cancers Google Scholar indexed journals neurological disorders articles neurological disorders Research articles neurological disorders review articles neurological disorders PubMed articles neurological disorders PubMed Central articles neurological disorders 2023 articles neurological disorders 2024 articles neurological disorders Scopus articles neurological disorders impact factor journals neurological disorders Scopus journals neurological disorders PubMed journals neurological disorders medical journals neurological disorders free journals neurological disorders best journals neurological disorders top journals neurological disorders free medical journals neurological disorders famous journals neurological disorders Google Scholar indexed journals elastography articles elastography Research articles elastography review articles elastography PubMed articles elastography PubMed Central articles elastography 2023 articles elastography 2024 articles elastography Scopus articles elastography impact factor journals elastography Scopus journals elastography PubMed journals elastography medical journals elastography free journals elastography best journals elastography top journals elastography free medical journals elastography famous journals elastography Google Scholar indexed journals tumours articles tumours Research articles tumours review articles tumours PubMed articles tumours PubMed Central articles tumours 2023 articles tumours 2024 articles tumours Scopus articles tumours impact factor journals tumours Scopus journals tumours PubMed journals tumours medical journals tumours free journals tumours best journals tumours top journals tumours free medical journals tumours famous journals tumours Google Scholar indexed journals biomarkers articles biomarkers Research articles biomarkers review articles biomarkers PubMed articles biomarkers PubMed Central articles biomarkers 2023 articles biomarkers 2024 articles biomarkers Scopus articles biomarkers impact factor journals biomarkers Scopus journals biomarkers PubMed journals biomarkers medical journals biomarkers free journals biomarkers best journals biomarkers top journals biomarkers free medical journals biomarkers famous journals biomarkers Google Scholar indexed journals
Article Details
1. Introduction
Ultrasonography (US) is a developed technology, which was in existence for more than 40 years. Yet, this field is not in a stagnant state because of the technical advancement are being made, constantly. The technological advances in this modality include the development of contrast agents, multidimensional imaging, and high-frequency imaging. Many terminologies used in US are introduced from the field of sonar and radar. For measuring blood flow, continuous-wave (CW) and pulsed-wave (PW) Doppler ultrasound devices became available. Duplex ultrasound scanners allow the imaging of anatomy and the measurement of blood flow with one single instrument [1]. Today, ultrasound is the second most utilized diagnostic imaging modality in medicine (second only to conventional x-ray) and is a critically important diagnostic tool of any medical facility [2]. Japanese investigators, Shigeo Satomura and YasuharaNimura, were the earliest contributors in the development of ultrasonic Doppler devices for monitoring tissue motion and blood flow in 1955 [3]. Another work which was concurrently going on in Japan and in the United States, IngeEdler and Hellmuth Hertz at the University of Lund in Sweden worked on echocardiography, an ultrasound imaging technique for imaging cardiac structures and monitoring cardiac functions [4]. In parallel with these developments in the diagnostic front, William Fry and his colleagues at the University of Illinois at Urbana worked on using a high-intensity ultrasound beam to treat neurological disorders in the brain [5]. This technique includes many imaging display modes, such as B-mode, M-mode, color power Doppler imaging, elastography for assessing tissue stiffness, harmonic imaging and contrast-enhanced ultrasound imaging for blood-filled spaces, specific agent-binding tissue or cell-expressed metabolites [6]. There are disease specific contrast agents developed to image particular target tissue by conjugating microbubbles with antibodies, peptides and other targeting moieties. Morphological changes in the tissue of interest are often considered as well-known sonography markers [7]. The scoring system of the morphology of the cyst includes its volume, surface, papillae, fragmentation, cyst wall thickness etc. Unfortunately, the morphologic changes alone do not allow for an exact finding of the pathological entity. However, to some extent, elastography and Doppler blood flow velocity helps to assess the tissue nature and used in monitoring therapy response. Measurements of tissue mechanical properties with ultrasound-elastography [8] and blood velocity using Doppler [9] can further characterize the tissue and helps to measure therapeutic response. These properties viz. , cellularity and vascularity are indicators of enhanced angiogenesis in anchored tumours [10]. However, the Doppler imaging is not sufficient to illustrate low velocity blood that flowing in very small blood vessels [11]. Hence, contrast agents are required to assess cancerous tumours with high sensitivity and specificity. There are numerous literature evidence showing the immense prospective utility of these contrast agents to assess blood velocity, relative volume and perfusion in tumour [12]. These biomarkers are not specific, and assessing specific molecular markers may be superior to understand the virulent nature of the tumours. Therefore, a new phase of micro-bubbles has been developed which are target-specific. In this review, the diagnosis and possible therapeutic approaches of cancer tumours (lumps) or cells using these targeted US contrast agents at different tissues are discussed.
2. Contrast Enhanced UltrasonographyImaging (CEUI)
Ultrasonography (US) has a broad range of applications, which can be further increased by using micro-bubbles as contrast agents (CAs). The US contrast agents (CAs) are small microspheres that are initially developed to better depict the cardiac chambers by well delineating the lumen and used to highlight the passage of blood in regions otherwise difficult to image, such as blood, which is nearly invisible to US without special processing. These CAs are microspheres filled with air or gases (perfluorocarbon), the shells are usually made of human serum albumin (HSA), lipids or surfactants. They act as blood cell tracers when they were injected into the venous system. These contrast agents in the blood improves the ability of US to follow the flow of blood in small vessels, and the blood perfusion in muscles, such as the heart, and facilitates identifying the walls of the moving chambers of the heart. For more than three decades, US contrast agents (UCA) were used clinically to enhance US imaging. When the UCAs are bonded by targeting ligands on their shell, they act as an ideal targeting UCA agent, which binds to the biomarkers expressed on intravascular endothelial cancer cells. The molecules, typically like protein, antibody, peptide or aptamer makes the UCAs as targeting functional moiety so that they can anchor on the selected biomarkers. The microspheres, after travelling in blood, diffuse and are released through the lungs by expiration. There are different types of micro-bubbles such as those of which filled with different gases, those with soft- or hard-shell materials, and in the range of nano- to micrometers [13]. These CAs are injected through intravascular mode and are facilitated for functional analyses. Specific contrast agents are developed by coupling specific ligands to the microbubbles’ shell, which attach to surface expressed marker molecules in the target of interest. These micro-bubbles can also be loaded with drugs or peptides, oligonucleotides, which can be destroyed by US pulses to release the entrapped agent near the target. Hyper-thermionic ablation of tumours is treated by US contrast agents that can increase cellular uptake of targeted drugs that are delivered locally by enhancing cellular membrane permeability [14]. Targeted contrast-enhanced US imaging (CEUSI) is an emerging imaging technique to assess biological alterations at the molecular level. Generally, vascular endothelial growth factor (VEGF). VEGF receptor 2 (VEGFR2) and endoglin (CD105) are the biomarkers which are targeted for tumour resulting from angiogenesis [15]. VEGFR2 is an extensively studied biomarker overexpressed on angiogenic vascular endothelial cells. In models associated with breast cancer, pancreatic adenocarcinoma and colon carcinoma, the visualization of cancer using VEGFR2 targeted micro-bubble are feasible using US [16].
3. UCAs for Breast Cancer
Ultrasonography imaging of the breast is gaining an important role in the evaluation of breast cancer due to technological development such as high-resolution ultrasound equipment in recent years. US is useful in the evaluation of many abnormalities seen in mammograms and can assist in the evaluation of palpable hidden masses. Furthermore, US imaging helps in differentiating cystic from solid breast masses, and can guide interventional diagnosis. It also provides valuable information about nature, the extent of the fibrous masses (Figure 1), lesions detection and characterization and in examining dense breast tissue [17]. An US contrast agent BR55; was functionalized using a lipopeptide that specifically targets VEGFR2. This functionalized US contrast agent was tested on breast tumour model in rats, where they specifically detected the presence of breast tumours bearing VEGFR2 receptor and was concluded that this BR55 US contrast agent will likely to be helpful in the earlier diagnosis breast cancer in humans [18]. Monoclonal antibody targeting VEGFR2 was conjugated with microbubbles and was used as targeted US contrast agents for the specific detection of breast cancer. This conjugate increased the US signal intensity as well as targeted the breast cancer tumours more specifically [19]. The use of B7-H3 targeted microbubble for US molecular imaging can be more selective in the detection of breast cancer when compared to VEGFR2 targeted microbubbles. B7-H3 (CD276) is a ligand for T-cell coregulatory receptor, which specifically expressed on the tumor vessels of breast cancer which can be used for targeted detection of breast cancers using US [20].
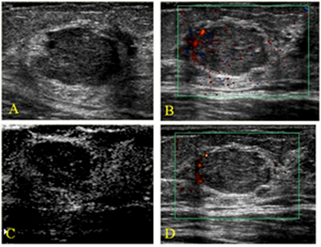
Figure 1: Non-cancerous tumour on the left breast (47 years) A. Gray-scale image showing irregular micro lobule mass (BI-RADS 4). B. Color Doppler image showing a peripheral regular artery, C. Contrast enhanced image (moderate peripheral enhancement is seen. D An enhanced colour Doppler image [21].
Phospholipid shelled nanobubbles were conjugated with monoclonal antibody Herceptin, which specifically targets breast cancer tumour expressing Her-2 biomarkers. In comparison with targeted and untargeted nanobubbles, the Herceptin-Nanobubble was very useful in molecular in vivo imaging and also had extended circulation time and increase tumour selectivity than untargeted nanobubbles [22]. Similarly, the monoclonal antibody Herceptin was conjugated with mesoporous silica nanoparticles (MSN). This MSN-Herceptin conjugates increases the US signal intensity as well inducing breast tumour specific cytotoxicity where Her-2 biomarkers were expressed [23]. In another study, polylactic acid, a biodegradable polymer was used to prepare microbubble and was functionalized with Herceptin. In an in vitro B-mode US imaging, this targeted US contrast agent improved the signal intensity along with the specific detection of Her2-positive breast cancer cells [24].
4. UCAs for Cervical and Ovarian Cancer
Uterine Cervical cancer is the most frequent gynaecological malignancy reported worldwide. It remains as one of the commonest cancer in women in developing countries of central and south America, parts of Africa and Southcentral Asia [25]. Etiological factors include exposure to Human Papillomavirus (types 16 and 18), other risk factors being the usage of oral contraceptive pills, smoking and lower social-economic status. Commonest histological types are Squamous cell carcinoma (85% of cases, Two third of cases), adenocarcinoma and adenosquamous carcinoma (10-25% cases). Clinical staging system such as pelvic exam, cystoscopy, rectoscopy underestimates or overestimate the actual extent of disease in several important staging parameters like the assessment of parametric and pelvic sidewall invasion, accurate measurement of tumour size and the evaluation of lymph node metastases. Imaging techniques are being increasingly used in the treatment of cervical cancer [26]. Though MRI and PET scan is being the first line diagnostic techniques, US has gained attention as imaging for cervical cancer. Trans-vaginal and the trans-rectal US gives detailed images for local staging of cervical cancer as the probe can be placed close to the tumour [27]. The sensitivity of trans-rectal US was proven to be higher than that of clinical exam in 124 women patients diagnosed with cervical cancer by Innocenti et al. TRUS sensitivity was 78% whereas clinical exam sensitivity was 50% [28]. Fischerova et al compared Transrectal US and MRI in early staged cervical cancer in a series of 95% cases [29]. TRUS was able to detect small cervical cancers (< 1cm) than MRI and its sensitivity was 83% versus MRI whose sensitivity was 50%. Testa et al proved sensitivity of TVUS higher in comparison with MRI in identifying parametrial involvement in cancer staging. Results were 60% for TVUS and 40% for MRI [30]. Epstein et al reported a European multicentre study comparing TVUS and MRI for delineating cervical tumour. TVUS was found to be superior to MRI in women with and without cone biopsy prior to surgery [31]. Fischerovahad described techniques for US evaluation of cervical cancer [32] which allows estimation of size and location of the tumor, depth of stromal infiltration, the involvement of parametrium, bladder and rectum and assessment of pelvic lymph nodes. Three dimensional US was found to be more accurate than two dimension in tumour volume estimation [33]. Ghi et al assessed the role of trans vaginal three dimensional US for local staging in 14 patients with early cervical cancer and the results agreed with histological data in 12 cases out of 14 cases [34]. Accuracies of Three dimensional US, MRI and clinical exam were compared by Byun et al. Accuracy was higher for 3D US (67%) compared to the clinical exam (62%) and MRI (41%) [35]. Angiogenesis is the production of new vessels in a specific area. It is essential for tumour growth and progression [36]. Specifically in cervical cancer angiogenesis has been shown to be an independent prognostic factor [37], Transvaginal doppler US allows in vivo non-invasive assessment of tumour angiogenesis [38]. Analyzing intra-tumoral vessels in cervical cancer was reported by Hsieh et al. in 1995 [39]. They found that 46. 2 % of cervical cancers exhibited blood flow color, Those patients with detectable colour signals lymph node involvement was more frequent as compared with those without colour signals detectable (33 % vs 5. 7 %, p = 0. 005). Cheng et al. reported a novel vascular index (VI) for the in vivo assessment of angiogenesis in patients with cervical carcinoma [40]. They evaluated 35 patients with stage Ib-IIa cervical carcinoma by transvaginal power Doppler US, and using image processor software they developed a vascular index (VI = number of colored pixels/number of total pixels) for each tumour. They found that the higher VI the higher tumoral stage, the deeper stromal invasion, the higher lymphovascular space invasion rate, and the higher pelvic lymph node metastases rate. In 2013, E-selectin specific US contrast agent was prepared by anchoring a peptide sequence on poly n-butyl cyanoacrylate stabilized microbubbles. The peptide sequence (IELLQAR) is specific towards E-selectin. This targeted microbubble has high specificity and increased US signal intensity in nude mice bearing human ovarian carcinoma xenografts [41]. In 2017, Epithelial ovarian cancer (EOC) is a gynaecologic malignancy, characterized by the over-expression of CA-125. Echogenic lipid nanobubbles functionalized with antibody targeting CA-125 were used for rapid detection of ovarian tumour. These targeted US nanobubbles have higher tumour accumulation, increased signal intensity in US and lesser wash-out rates. Thus, CA-125 antibody conjugated nanobubbles may be helpful for improved diagnosis of ovarian cancer using US molecular imaging. The detection of ovarian cancer by targeted US contrast agent was reported by Deshpande N et al. They have used monoclonal antibody against αvβ3integrin and endoglin for specific detection of ovarian tumours, thereby anchoring those antibodies on microbubbles for targeted US imaging [19]. An attempt was made to demonstrate the capability of dual-targeted microbubbles against single targeted microbubbles. The perfluorocarbon filled microbubbles were conjugated with monoclonal antibodies against VEGFR2 and αvβ3 integrin that specifically targets ovarian cancer. This research concludes that the signal intensity from dual-targeted microbubbles was significantly higher than signal intensity from single targeted microbubbles [42].
5. UCAs for Liver Cancer
Although, normal B-mode sonography is the widely available screening of liver disease it is less accurate when compared to CT and MRI for the detection of small lesions. However, with the new invention of contrast agents, contrast enhanced US (CEUS) plays a vital role in the initial diagnosis and real-time biopsies for detection and staging of tumors in the liver and the precise targeting of the lesion. When pathological diagnosis required, US is the imaging technique widely used worldwide to guide liver biopsy, and CEUS can guide focal biopsy by increasing accuracy rates in the case of larger focal liver lesions [43]. Liver cancers are the third most common cause of cancer related mortality. Most common malignancy of liver in adults is hepatocellular carcinoma (HCC) [44]. Early detection is crucial for therapeutic interventions and US is the most widely accepted and used imaging technique. In US imaging, HCC appears as iso-echoic or hyper-echoic lesion. In clinical practice, percutaneous biopsy are generally operated under US or CT guidance. However, radiation exposure limits the utility of CT-guided percutaneous treatment [45]. Solbiati et al reported that with the help of CEUS, the rate of partial necrosis has been significantly reduced in real-time management of ablations [46]. In this study sulphur hexafluoride micro-bubbles (SonoVueâ) was used as a contrast agent before, during and after the radio-frequency ablation procedure. Generally, the liver takes-up some of the micro bubbles, during liver-phase that lasts for 30 minutes to a few hours. During this period, the liver can be better visualised in the microbubble-specific (harmonic) imaging mode [47]. Many liver lesions such as metastases, hepatocellar carcinoma, are greatly enhanced with microbubbles (Figure-2). USCA is safe to use with minimal side-effects and also safe to permit repeated administration in the same session.
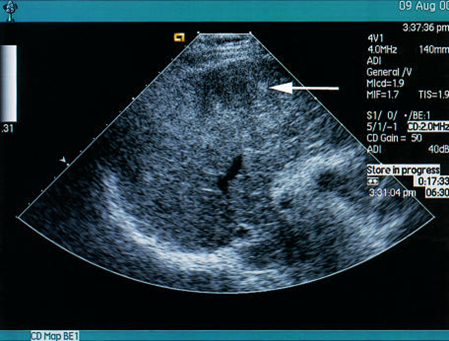
Figure 2: Liver specific micro-bubbles (hepatacellular carcinoma (Top- poorly shown lesion, bottom image shows the liver specific contrast agent (Levovist)). The defects represent the foci of hepatocellular carcinoma [48].
6. UCAs for Prostate Cancer
Ultrasonography of the prostate uses sound waves to produce pictures of a man’s prostate gland and to help diagnose symptoms such as difficulty urinating or an elevated blood test result. It’s also used to investigate a nodule found during a rectal exam, detect abnormalities and determine whether the gland is enlarged. Prostate US also called trans-rectal US, provides images of a man’s prostate gland and surrounded tissue. The examination typically requires the insertion of an US probe into the rectum of the patient. The probe sends and receives sound waves through the wall of the rectum into the prostate gland, which is situated right in front of the rectum. The focused US is a non-invasive radiation free method to destroy prostate tissue and treat prostate diseases. Using real tie image guidance the physician directs a focused beam of US energy to a selected volume in the patient’s prostate gland. The energy heats and destroys the targeted tissue at the focal point within seconds. This process is repeated until the entire selected volume or the entire gland is destroyed. The technology has been successfully used to treat a wide variety of prostate related diagnosis, including benign prostatic hyperplasia (BPH) and prostate cancer. Focal prostate gland ablation or diffuse prostate gland ablation is possible depending on the clinical scenario. More than 50,000 men around the world have been treated with focused US for prostate cancer. It is the leading clinical applications of the technology, more than 50% of all focused US procedure performed to date were prostate cancer. Fluorescein isothiocyanate and prostate stem cell antigen (PSCA) monoclonal antibody were conjugated with polyethyleneimine containing carbon nanotubes. After in vivo experiments, it was found that these conjugates have a potential use as a contrast agent in US imaging application. Also, this conjugate specifically targets prostate cancer in mice model and acts as a multifunctional agent for US imaging and cancer drug delivery. Microbubbles derived from biodegradable PLGA-PEG polymer was conjugated with urea based PSMA inhibitor. These targeted microbubbles were investigated for targeting efficiency and US imaging capability for the specific diagnosis of prostate cancer [49]. In another study, PSMA monoclonal antibody was conjugated with microbubbles prepared using biotin-avidin technology. This targeted microbubble can target and bind to prostate cancer and enhance the US contrast thereby paving the way for early diagnosis of prostate cancer [50]. Assessing some parameters such as arrival time, peak time, peak intensity and enhanced duration as imaging indicators helps to compare different cancer type and targeted versus blank nano-bubbles (NBs). For instance, in the prostate cancer xenografts (LNCap and C4-2) the peak intensity values (P=0. 003 and 0. 002 respectively) were significantly higher and enhanced durations were significantly longer (P=0. 001 and 0. 004 respectively) in the targeted NBs than in the blank NBs (Figure 3).
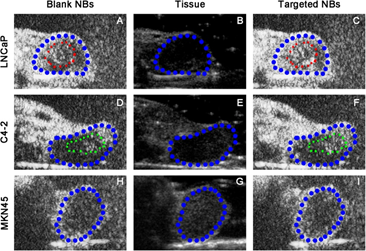
Figure 3: Targeted nano-bubbles (NBs) in tumour bearing nude mice.
A and D shows blank NBs; B and E are B-mode US images; C and F show targeted NBs (TNBs). Where, LNcap and C4-2 are two kinds of animal prostate adenocarcinoma xenografts.
7. Summary
Ultrasonography gained its importance in recent years due to many advantages such as low imaging and equipment cost, wide availability, non-invasive, portability etc. Molecular imaging using US is rapidly gaining its importance with the invention of numerous contrast-enhanced US agents with high attracting targeting ligands. High spatial and temporal resolution, non-invasive nature of imaging, real-time imaging, lack of ionising radiation are added advantage for this imaging mode. Recent technological development and sophisticated instrumentation facilitate this imaging modality to its wide reach among the research and clinical community. However, in respect of the use of targeted microbubbles, this field is currently limited to pre-clinical research. This may be due to high-priced and immunogenic reactive property, allergic reactions of contrast agents are further limiting its application in clinical settings. Furthermore, the US images with low-contrast resolution have difficult in distinguishing tumour from the surrounding tissue, which depends on the operator skill and a risk of subjective variation while interpreting the image. Other limitations of US include variable attenuation by body tissues, inability for imaging lung, small field of view in some 2-dimensional probes etc.
8. Conclusion
US has made remarkable progresses in the last decades because of various advancement in this field and technology progress. The advancement of imaging procedures is anticipated to support the clinical management of patients. CEUS is proved to be of great value in the management of various disease conditions with integral benefits, such as sufficient high safety for patients those who are allergic to iodine and kidney failures, and also it is free from ionizing radiation, apart from these, stress-free repeatability and it also offers a high temporal resolution. The incredible application of CEUS to diagnostic and therapeutic application for various diseases provides more chances of disease management in different pathophysiological stages.
Acknowledgment
The authors would like to thank Director-Research, Principal-Faculty of Allied Health Sciences, Chettinad Academy of Research and Education for their valuable support and guidance.
References
- Shung KK. Diagnostic ultrasound: Imaging and blood flow measurements: CRC press (2015).
- Wolbarst AB. Looking within: how X-ray, CT, MRI, ultrasound, and other medical images are created, and how they help physicians save lives: Univ of California Press (1999).
- Shung KK. Diagnostic ultrasound: Past, present, and future. J Med Biol Eng 31 (2011): 371-374.
- Shung KK. DIAGNOSTIC ULTRASOUND (2006).
- O’Brien Jr WD, Dunn F. high-intensity focused ultrasound. Physics Today 68 (2015): 40.
- Feleppa EJ, Mamou J, Porter CR, et al. Quantitative ultrasound in cancer imaging. Seminars in oncology 38 (2011): 136-150.
- Tabor A, Jensen FR, Bock JE, et al. Feasibility study of a randomised trial of ovarian cancer screening. Journal of medical screening 1 (1994): 215-219.
- Thittai AK, Yamal J-M, Mobbs LM, et al. AXIAL-SHEAR STRAIN ELASTOGRAPHY FOR BREAST LESION CLASSIFICATION: FURTHER RESULTS FROM IN VIVO DATA. Ultrasound in medicine & biology 37 (2011): 189-197.
- Goertz DE, Yu JL, Kerbel RS, et al. High-frequency Doppler ultrasound monitors the effects of antivascular therapy on tumor blood flow. Cancer research 62 (2002): 6371-6375.
- Folkman J. Role of angiogenesis in tumor growth and metastasis. Seminars in oncology 29 (2002): 15-18.
- Delorme S, Peschke P, Zuna I, et al. [Imaging the smallest tumor vessels using color Doppler ultrasound in an experiment]. Der Radiologe 41 (2001): 168-172.
- Lassau N, Chami L, Benatsou B, et al. Dynamic contrast-enhanced ultrasonography (DCE-US) with quantification of tumor perfusion: a new diagnostic tool to evaluate the early effects of antiangiogenic treatment. European radiology 6 (2007): F89-F98.
- Paefgen V, Doleschel D, Kiessling F. Evolution of contrast agents for ultrasound imaging and ultrasound-mediated drug delivery. Frontiers in Pharmacology 6 (2015): 197.
- Kiessling F, Fokong S, Koczera P, et al. Ultrasound microbubbles for molecular diagnosis, therapy, and theranostics. Journal of nuclear medicine : official publication, Society of Nuclear Medicine 53 (2012): 345-348.
- Alzaraa A, Gravante G, Chung WY, et al. Targeted microbubbles in the experimental and clinical setting. The American Journal of Surgery 204 (2012): 355-366.
- Anderson CR, Rychak JJ, Backer M, et al. scVEGF microbubble ultrasound contrast agents: a novel probe for ultrasound molecular imaging of tumor angiogenesis. Invest Radiol 45 (2010): 579-585.
- Madjar H. Role of Breast Ultrasound for the Detection and Differentiation of Breast Lesions. Breast Care 5 (2010): 109-114.
- Pochon S, Tardy I, Bussat P, et al. BR55: a lipopeptide-based VEGFR2-targeted ultrasound contrast agent for molecular imaging of angiogenesis. Investigative radiology 45 (2010): 89-95.
- Deshpande N, Ren Y, Foygel K, et al. Tumor Angiogenic Marker Expression Levels during Tumor Growth: Longitudinal Assessment with Molecularly Targeted Microbubbles and US Imaging. Radiology 258 (2011): 804-811.
- Bachawal SV, Jensen KC, Wilson KE, et al. Breast Cancer Detection by B7-H3-Targeted Ultrasound Molecular Imaging. Cancer research 75 (2015): 2501-2509.
- Stanzani D, Chala LF, de Barros N, et al. Can Doppler or contrast-enhanced ultrasound analysis add diagnostically important information about the nature of breast lesions? Clinics 69 (2014): 87-92.
- Jiang Q, Hao S, Xiao X, et al. Production and characterization of a novel long-acting Herceptin-targeted nanobubble contrast agent specific for Her-2-positive breast cancers. Breast Cancer (Tokyo, Japan) 23 (2016): 445-455.
- Milgroom A, Intrator M, Madhavan K, et al. Mesoporous silica nanoparticles as a breast-cancer targeting ultrasound contrast agent. Colloids and surfaces B, Biointerfaces 116 (2014): 652-657.
- Jun L, Jie L, Thomas JR, et al. Biodegradable nanoparticles for targeted ultrasound imaging of breast cancer cells in vitro. Physics in Medicine & Biology 52 (2007): 4739.
- Maddams J, Utley M, Møller H. Projections of cancer prevalence in the United Kingdom, 2010–2040. British journal of cancer 107 (2012): 1195-1202.
- Delgado G, Bundy B, Zaino R, et al. Prospective surgical-pathological study of disease-free interval in patients with stage IB squamous cell carcinoma of the cervix: a Gynecologic Oncology Group study Gynecologic oncology 38 (1990): 352-357.
- Testa AC, Di Legge A, De Blasis I, et al. Imaging techniques for the evaluation of cervical cancer. Best Practice & Research Clinical Obstetrics & Gynaecology 28 (2014): 741-768.
- Innocenti P, Pulli F, Savino L, et al. Staging of cervical cancer: reliability of transrectal US. Radiology 185 (1992): 201-205.
- Fischerova D, Cibula D, Stenhova H, et al. Transrectal ultrasound and magnetic resonance imaging in staging of early cervical cancer. International journal of gynecological cancer: official journal of the International Gynecological Cancer Society 18 (2008): 766-772.
- Testa AC, Ludovisi M, Manfredi R, et al. Transvaginal ultrasonography and magnetic resonance imaging for assessment of presence, size and extent of invasive cervical cancer. Ultrasound in obstetrics & gynecology: the official journal of the International Society of Ultrasound in Obstetrics and Gynecology 34 (2009): 335-344.
- Epstein E, Testa A, Gaurilcikas A, et al. Early-stage cervical cancer: Tumor delineation by magnetic resonance imaging and ultrasound — A European multicenter trial. Gynecologic oncology 128 (2013): 449-453.
- Fischerova D. Ultrasound scanning of the pelvis and abdomen for staging of gynecological tumors: a review. Ultrasound in obstetrics & gynecology: the official journal of the International Society of Ultrasound in Obstetrics and Gynecology 38 (2011): 246-266.
- Chou C-Y, Hsu K-F, Wang S-T, et al. Accuracy of Three-Dimensional Ultrasonography in Volume Estimation of Cervical Carcinoma. Gynecologic oncology 66 (1997): 89-93.
- Ghi T, Giunchi S, Kuleva M, et al. Three-dimensional transvaginal sonography in local staging of cervical carcinoma: description of a novel technique and preliminary results. Ultrasound in Obstetrics and Gynecology 30 (2007): 778-782.
- Byun JM, Kim YN, Jeong DH, et al. Three-dimensional transvaginal ultrasonography for locally advanced cervical cancer. International journal of gynecological cancer : official journal of the International Gynecological Cancer Society 23 (2013): 1459-1464.
- Folkman J, Watson K, Ingber D, et al. Induction of angiogenesis during the transition from hyperplasia to neoplasia. Nature 339 (1989): 58-61.
- Abulafia O, Sherer DM. Angiogenesis in the uterine cervix. International journal of gynecological cancer : official journal of the International Gynecological Cancer Society 10 (2000): 349-357.
- Cosgrove D. Angiogenesis imaging--ultrasound. The British journal of radiology 76 (2003): S43-S49.
- Hsieh CY, Wu CC, Chen TM, et al. Clinical significance of intratumoral blood flow in cervical carcinoma assessed by color Doppler ultrasound. Cancer 75 (1995): 2518-2522.
- Cheng WF, Lee CN, Chu JS, et al. Vascularity index as a novel parameter for the in vivo assessment of angiogenesis in patients with cervical carcinoma. Cancer 85 (1999): 651-657.
- Fokong S, Fragoso A, Rix A, et al. Ultrasound molecular imaging of E-selectin in tumor vessels using poly n-butyl cyanoacrylate microbubbles covalently coupled to a short targeting peptide. Invest Radiol 48 (2013): 843-850.
- Willmann JK, Lutz AM, Paulmurugan R, et al. Dual-targeted Contrast Agent for US Assessment of Tumor Angiogenesis in Vivo. Radiology 248 (2008): 936-944.
- Wu W, Chen MH, Yin SS, et al. The role of contrast-enhanced sonography of focal liver lesions before percutaneous biopsy. AJR American journal of roentgenology 187 (2006): 752-761.
- Ferlay J, Soerjomataram I, Dikshit R, et al. Cancer incidence and mortality worldwide: sources, methods and major patterns in GLOBOCAN 2012. International journal of cancer 136 (2015): E359-E386.
- Maruyama H, Yoshikawa M, Yokosuka O. Current role of ultrasound for the management of hepatocellular carcinoma. World J Gastroenterol 14 (2008): 1710-1719.
- Solbiati L, Ierace T, Tonolini M, et al. Guidance and monitoring of radiofrequency liver tumor ablation with contrast-enhanced ultrasound. European journal of radiology 51 (2004): S19-S23.
- Forsberg F, Goldberg BB, Liu JB, et al. Tissue-specific US contrast agent for evaluation of hepatic and splenic parenchyma. Radiology 210 (1999): 125-132.
- Blomley MJK, Cooke JC, Unger EC, et al. Microbubble contrast agents: a new era in ultrasound. BMJ: British Medical Journal 322 (2001): 1222-1225.
- Sanna V, Pintus G, Bandiera P, et al. Development of Polymeric Microbubbles Targeted to Prostate-Specific Membrane Antigen as Prototype of Novel Ultrasound Contrast Agents. Molecular Pharmaceutics 8 (2011): 748-757.
- Wang L, Li L, Guo Y, et al. Construction and In Vitro/In Vivo Targeting of PSMA-Targeted Nanoscale Microbubbles in Prostate Cancer. The Prostate 73 (2013): 1147-1158.